Understanding Dalton`s Law in Physics IDC DiveTheory
- Tracy Gunn
- May 18, 2021
- 10 min read
Updated: Jan 11, 2024
Dalton's Law (also called Dalton's Law of partial pressures) states that "The total pressure exerted by a mixture of gases is equal to the sum of the pressures of each of the different gases making up the mixture – each gas acting as if it alone were present and occupied the total volume."
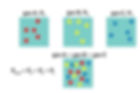
The sum of all the gases will always equal a whole (100%)
As the pressure increases, the Partial Pressure (NOT THE PERCENTAGE, THE PERCENTAGE NEVER CHANGES) will increase.
John Dalton
John Dalton was an English Chemist born 1766 - 1844. He is best known for his atomic theory, but he is also renowned for his research into colour blindness, which he and his brother suffered from. He formulated the theory of partial pressure in 1801, and its applications to diving are wide and extremely important, especially in technical diving.
While Archimedes' Principle, Charles's Law and Boyle's Law focus on the physical effect, Henry's Law and Dalton's Law explain the physiological effect.
Daltons' Law of partial pressure enables us to predict how much of a specific gas is dissolved into the blood at a given depth. His Law relates directly to physiology and describes the behaviours of any gas in a mixture of gases. This is important because of each individual gas's physiological effect on a diver when the partial pressure rises above a particular level.
At higher partial pressures, gases in the blood alter the electrical properties in the cerebral cell membranes and may cause narcotic anaesthetic, nitrogen narcosis or toxicity in the body.
Understanding this Law of partial pressure helps determine air supply, gas volumes, and maximum depth when diving with enriched air; it helps us plan our dives, determine the ideal gas mixture for the type of diving we wish to do and when to change gases underwater. It also helps us avoid problems with individual gases under pressure within a mixture.
Henry's Law and Dalton's Law are used to determine decompression models used in dive tables and computers.
Gas toxicity when diving
Nitrogen, oxygen, and carbon monoxide can all have toxic or narcotic effects during diving.
Nitrogen Narcosis occurs when the partial pressure of nitrogen (PN2) is 2.37 - 3.16 bar (20 to 30 + m) and above absolute breathing air and is caused by the partial pressure of nitrogen in the blood.
Oxygen toxicity happens when partial pressures of oxygen exceed 1.4 - 1.6 bar and above. (56-67 + msw). More of a concern when technical diving or using EANx than when diving with air within recreational limits
Small amounts of carbon monoxide in your breathing air that are harmless at the surface can become toxic at depth, causing carbon monoxide poisoning.
High-pressure nervous syndrome can occur when divers are deeper than 150-180 meters (500-600 ft). Especially when the dive is rapid and the diver is breathing helium and oxygen mixes. Like Nitrogen narcosis, it resolves itself when the diver ascends or the rate of descent is slowed. It can be avoided by adding a small amount of a narcotic gas, like nitrogen, to the mix.
Daltons Law
Basically, the Law can be divided into 3 categories.
- Percentage (hint-it never changes)
- Partial pressure (expressed in decimal. Think partial-decimal)
- Surface equivalent
Percentage

Boyles Law explains that when breathing air at depth, each breath contains more molecules than at the surface (density directly proportional to pressure). This means that at depth, we breathe the same percentage of each gas in a mixture as we would at the surface, but the number of molecules we breathe increases with pressure.
From this, we can show that - as the pressure increases, the Partial Pressure (NOT THE PERCENTAGE, THE PERCENTAGE NEVER CHANGES) will increase.
e.g., 21% Oxygen + 79% nitrogen =100% Air is the same at every depth.
The physiological effect of a gas changes as partial pressure changes, as does the surface equivalent, but the actual percentage remains the same.
Don't get caught out with this simple fact in the exams.
Partial Pressure

Each gas within a mixture exerts its own individual pressure, acting as if it alone were present and occupied the total volume. This is called partial pressure (abbreviated "pp" or "P" before a gas such as "PO2" for "partial pressure of oxygen") as it is part of the total pressure exerted by that gas mixture and is proportional to the number of molecules of that particular gas within the gas mixture. Instead of counting molecules, Dalton's Law says we can use partial pressures.
If you take a gas underwater, the pressure increases with depth. The partial pressure of each gas in the mix also increases proportional to its fraction in the mix.
You can determine the partial pressure by simply multiplying the gas percentage (in decimal) in the mix by the total absolute pressure.
Remember partial- decimal.
Examples using air. Let's assume 21% oxygen and 79% nitrogen and ignore other gases.
21% is expressed as 0.21 (21÷100=0.21 - this is % expressed in decimal form)
Surface = 1 bar/ata
PO2 = 0.21 ata oxygen × 1 ata = 0.21 oxygen
PN2 = 0.79 ata nitrogen × 1 ata = 0.79 nitrogen
1 ata
* note that adding the partial pressure will always equal the surrounding pressure. In this case: - 1 ata (0.21 + 0.79 = 1)
40msw (meters sea water) = 5 ata (40÷10+1=5 ata)
PO2= 0.21 ata oxygen × 5 ata = 1.05 ata oxygen *
PN2= 0.79 ata nitrogen × 5 ata = 3.95 ata nitrogen
5 ata
* note that PO2 of 1.05 ata is basically the same as breathing pure oxygen (100%) at the surface
This means that the physiological effect of oxygen is the same whether breathing pure oxygen (100%) at the surface or breathing air (21%) at 40 meters. The individual gases' physiological effect depends on their partial pressure. Although other gases may be present and have their own effect, it is immaterial to the other gases in the mix.
This is important to calculate the partial pressure of gases to any depth. Doing this allows you to see when oxygen reaches high levels that may cause oxygen toxicity.
For example, oxygen becomes toxic when it exceeds 1.4 - 1.6 bar/ata
Imagine diving with air to 60 m
60msw = 7 ata (60÷10+1=7 ata)
PO2= 0.21 ata oxygen × 7 ata = 1.47 ata oxygen *
PN2= 0.79 ata nitrogen × 7 ata = 5.53 ata nitrogen
7 ata
* Possible O2 toxicity
So, although we won't reach this depth in recreational diving, this becomes an issue when using enriched air (EANx) with blends of oxygen-nitrogen that use higher oxygen content than normal air.
Example - using a blend of 36% EANx to 30 msw
30msw = 4 ata (30÷10+1=4 Bar)
PO2 = 0.36 oxygen × 4 ata = 1.44 bar/ata
Because of this, different blends of EANx have different maximum depths depending upon the depth that you reach PO2 of 1.4 -1.6 bar/ata
In technical diving, the body can reach 1.4 bar/ata of PO2 when the diver is active or 1.6 bar/ata when resting (decompression stop), so even air has too much oxygen when below 67 meters.
Example - Air at 67msw
67m= 7.7 ata (67÷10+1=7.7 ata)
PO2 = 0.21 ata oxygen× 7.7 ata = 1.6 bar/ata
Tec divers also carry two or more different gas blends for decompression, and each has its own oxygen content and maximum depth based on the PO2. Air is often diluted with helium to reduce oxygen partial pressure and narcosis.
All gases that can be breathed, with the exception of helium and possibly neon, have a narcotic effect, although the exact reason for this phenomenon is not clearly understood.
We know that as the depth increases, mental impairment becomes a hazard.
Example - pure oxygen at 6 msw
6msw = 1.6 bar/ata (6÷10+1=1.6 bar/ata)
PO2= 1 × 1.6 bar = 1.6 bar/ata
* note - perfect for off-gassing
Technical divers breathe pure oxygen on decompression stops of 6mt and 3mt. It's not necessarily the presence of oxygen but the total absence of any inert gases, which speeds up off-gassing. This allows nitrogen to diffuse out of the body more rapidly. Also, any inert gas bubbles in the circulatory system will most likely shrink or disappear before becoming a problem.
Surface Equivalency
The body responds to the gas you breathe based on its partial pressure - not on the percentage of the gas in the mix. This means that as pressure increases, so does the physiological effect.
The Surface Equivalent is the fraction of gas you would have to breathe at the surface to have the same effect at a particular depth.
To find the surface equivalent, you may use the "pp" of the gas at depth as the fraction of the gas at the surface. (i.e., 0.21 bar/ata is 21%)
If the "pp" exceeds 1.0 ata, there can be no surface equivalent because the partial pressure would exceed the total pressure available at the surface (surface pressure is 1 ata. It is impossible to be more than this naturally, hence no surface equivalent).
Dalton's Law becomes significant when you look at impurities in the air we breathe. Surface equivalency applies when comparing the effects of a single gas breathed at a particular depth with the effects of a greater percentage of the same gas at the surface. It is of concern when dealing with toxic contaminants in our breathing gases.
Carbon monoxide (CO) is a product of combustion. It can enter a diver's air if the lubricating oil in a malfunctioning compressor becomes hot enough to partially combust or the intake valve of the compressor is too close to the engine exhaust. In small amounts, it is not toxic at the surface, but breathing higher partial pressures of CO at depth may be deadly.
Example - A compressor fills a scuba tank with 0.5% carbon monoxide (CO) in the breathing air. This is not toxic at the surface, but look at what happens when that tank is taken down to 40 msw.
40msw = 5 ata (40÷10+1= 5 bar/ata)
0.5% in decimal is .005 (0.5÷100=.005)
PCO = .005 ata × 5 ata= .025 bar/ata
.025 bar/ata = 2.5% surface equivalent
you could also multiply the percentage
5% × 5 bar/ata = 2.5% surface equivalent
So, even though 0.5% at the surface is minimal and not likely to be toxic, it is a different story at 40 msw, where the PCO is .025 bar/ata, the surface equivalent of breathing 2.5% CO- a poisonous level. The percentage of CO in the tank did not change, but the physiological effect did when the partial pressure increased.
Example - Would CNS (Central Nervous System) oxygen toxicity ever be likely breathing ANY gas mixture with oxygen at the surface? Remember that CNS toxicity is only expected when partial pressure exceeds 1.4 ata.
No, the highest fraction of oxygen that you can have is 100% at the surface. This is a PO2 of 1 ata - the highest partial pressure you can have at the surface. So, you could not reach a PO2 of 1.4 ata at the surface, only at depth.
Other formulas that use Daltons Law

The bottom of the triangle multiplies with each other to find the top missing value.
The top divides the bottom to find the other missing bottom value.
What is the PO2 if we wish to dive to 40 msw (40÷10+1=5 ata) on air?
PO2=0.21 (oxygen) × 5 ata = 1.05 bar/ata
What if we want to know at what absolute pressure we reach PO2 of 1.6 bar/ata using 36% O2?
1.6 ata ÷ 0.36 ata = 4.44 ata (approx. 34 msw)
What percentage of oxygen can we use if we wish to dive to 50 msw (50÷10+1=6 ata) and not exceed 1.4 bar/ata?
1.4 ata÷ 6 ata =0.23 bar/ata or 23% oxygen
Daltons Law and Nitrox (EANx)

Don't worry, you won't get these in the exams, but you might want to know them if you become a Nitrox instructor.
Altitude
At altitude, partial pressures are lower than at sea level and, as such, have fewer oxygen molecules per volume. At the summit of Mt Everest, even though the air still contains 21% oxygen and 78% nitrogen (the percentage does not change), the number of molecules per air volume is around 1/3 that at sea level. As such, the partial pressures are also only 1/3. This lack of oxygen volume can cause altitude sickness.
In diving, this change in partial pressure becomes important when we look at decompression theory and dissolved nitrogen in our body. The rate at which this inert gas can be safely released from our body upon ascent is greatly affected by the lowered partial pressures of altitude. Managing this pressure gradient is key to managing decompression risk with regard to safe ascent rates. At altitude, the release of nitrogen is much faster than at sea level due to the lowered partial pressures. As such, exceeding a safe pressure gradient can easily occur, causing decompression sickness. The use of special tables and procedures is required.
We will discuss this further in Henry's Law.
The equations
Daltons' Law can always have the same equation viewed 3 ways
The percentage of the gas (never changes)
The partial pressure (expressed in decimal. Think partial…decimal)
Surface Equivalent - The equivalent of breathing "X" percentage at the surface (expressed in %)
Example 1: Enriched Air 36% Oxygen at 40m (5bar)
1 Percentage (never changes)
The percentage at 40mt will still be the same
Oxygen 36%, Nitrogen 64%
Remember, the % does not change; only the "pp" and surface equivalent
2 Partial pressure (partial-decimal)
PO2= 0.36 x 5 bar =1.8 bar/ata * oxygen toxicity in this range
PN2= 0.64 x 5 bar =3.2 bar/ata
5 Bar
3. Surface equivalent
1.8 bar/ata oxygen (=180%)
3.2 bar/ata nitrogen (=320%)
*note. The ata is greater than 1, so there can be no real surface equivalent
or
Oxygen Surface Equivalent 36% X 5 = 180%
Nitrogen Surface Equivalent 64% X 5 = 320%
* Impossible at the surface, only under pressure
Example 2: Carbon monoxide 1% at 50 msw (6 bar/ata)
1. Percentage (never changes)
The percentage at 50msw will still be the same
Carbon monoxide CO is 1%
Remember, the % does not change; only the "pp" and surface equivalent
2. Partial pressure (partial-decimal)
PCO= .01 × 6 bar = .06 bar/ata
3. Surface equivalent
.06 bar/ata carbon monoxide (=6%)
or
Carbon monoxide Surface Equivalent 1% × 6 bar = 6%
* poisonous at this level
Now you are ready for some testing on Dalton's law
Try the exam below.
Here are links to all the physics blogs
And to all the exams

Water, Heat, Light, Sound, and Gases Exam

Archimedes' Exam part 1

Archimedes' Exam Part 2

Under Pressure Exam

Boyle's Law Exam Part 1
Single-Level Depth Changes

Boyle's Law Exam Part 2
Multi-Level Depth Changes

Charles' Law Exam

Henry's Law Exam

Dalton's Law Exam
(1) Goodwin, M. (2007). Now Take a Deep Breath. Ocean Explorer.NOAA.Gov. https://oceanexplorer.noaa.gov/edu/lessonplans/breath.pdf
(2) M. (2014, August 2). Dalton's Law. Divers Who Want To Learn More. https://diverswhowanttolearnmore.wordpress.com/2014/08/04/daltons-law/
(3) Merck Manuals. (2021). Gas Toxicity During Diving. Merck Manuals Professional Edition. https://www.merckmanuals.com/professional/injuries-poisoning/injury-during-diving-or-work-in-compressed-air/gas-toxicity-during-diving
(4) The Encyclopedia of Recreational Diving (3rd ed.). (2008). PADI.
(5) Divemaster Course Instructor Guide (1999 edition). (2005). PADI.
(6) Inc, E. (2020, December 15). A Closer Look at Gas Laws, Hypoxia and Altitude. Environics. https://www.environics.com/2011/09/30/environics-post-bid-45220-a-closer-look-at-gas-laws-hypoxia-and-altitude/
(7) An Explanation of Pressure and the Laws of Boyle, Charles, Dalton, and Henry. (1997). Scuba Diving Explained. http://www.lakesidepress.com/pulmonary/books/scuba/sectiond.htm
(8) Physics. (2019). NOAA Diving Manual - Physics of Diving. Published. https://www.ehs.ucsb.edu/files/docs/ds/physics.pdf